The Role of Spike Protein in Heart Inflammation and Blood Clotting After COVID-19 Vaccination: A Deeper Dive
In this series, “Promise or Peril: Alarming COVID-19 mRNA Vaccine Issues,” we explore how the introduction of mRNA technology lacked an adequate regulatory framework, setting the stage for serious adverse events and other concerns related to inadequate safety testing of lipid nanoparticles, spike protein, and residual DNA- and lipid-related impurities, as well as truncated/modified mRNA species.
Previously: In Part 1, we introduced how the U.S. Food and Drug Administration (FDA) relaxed the rules for mRNA vaccines compared to mRNA therapies and discussed the available data regarding LNP distribution throughout the body based on animal testing, the fact that human testing was not done, and the lack of mRNA or spike protein biodistribution data. In Parts 2 and 3, we explored how the LNPs are constructed and how they behave in the body and affect health.
Now we turn to another problem—the cargo contained in the LNP capsules: the mRNA and its encoded spike protein. We introduce the inflammatory response to the spike protein and one of its subunit proteins and how they may contribute to serious adverse events such as myocarditis and blood clotting.
Rochelle Walensky, former director of the U.S. Centers for Disease Control and Prevention (CDC), stated on “Good Morning America” in June 2021 that myocarditis cases are “really quite rare … minor, self-limited, they generally resolve with rest and standard medications.” However, this assertion was made based on a preliminary review of 300 cases and before conducting long-term follow-up.
A study published on Aug. 1 followed 40 adolescents in Hong Kong for up to a year. Follow-up testing performed in 26 patients with initial abnormal findings revealed that 58 percent of those with vaccine-associated myocarditis had persistent heart muscle scarring. The authors concluded: “There exists a potential long-term effect on exercise capacity and cardiac functional reserve during stress.”
This series demonstrates how exposure to the spike protein results in downstream cardiovascular issues. Given that vaccination causes the body to produce more spike protein, it is clear that additional research was needed to understand the health impacts of vaccination prior to licensure.
Summary of Key Facts
- The SARS-CoV-2 spike protein and its S1 subunit have known impacts on the cardiovascular system, such as an increased risk of blood clotting.
- The vaccine-induced spike protein and its S1 subunit have been found in the blood following vaccination.
- In lab studies, the spike protein activates white blood cells and may trigger an inflammatory response or clotting.
- Free spike protein was found in the blood of adolescents and young adults with post-mRNA vaccine myocarditis but not in healthy control subjects without myocarditis.
- The S1 subunit can interact with ACE2, platelets, and fibrin and may be what leads to an inflammatory response driving serious adverse events, including clots, myocarditis, and neurological problems.
- As discussed in Part 3, lipid nanoparticles (LNPs) act as adjuvants, stimulating the immune system. This innate immune response peaks within six hours of vaccination and returns to baseline by about day nine, temporally corresponding to the onset of myocarditis, which typically occurs within the first seven days following mRNA COVID-19 vaccination.
- Studies have not been done to evaluate how vaccination affects those who have already been infected with SARS-CoV-2.
- The spike protein was implicated in small vessel microclots during COVID-19 illness; thus, postvaccination cardiovascular effects should have been anticipated.
- The first deadline for FDA-mandated post-authorization safety studies has passed, yet to the best of our knowledge, the full report has not been made available to the public.
The spike protein protrudes from the SARS-CoV-2 virus like a crown of sticky handles. The job of the spike protein is to grab onto the ACE2 receptor so the virus can enter the cell. The ACE2 receptor is found in many human cells in the lungs, kidneys, gut, heart, and the lining of the blood vessels.
Spike protein is comprised of two parts: the S1 and S2 subunits. The S1 subunit protein sits at the tip of the spike protein and is responsible for attaching to the ACE2 receptor. Once bound to the receptor, the spike protein changes shape to allow the virus to enter. Having accessed the inside of the cell, the SARS-CoV-2 virus uses the cell’s own protein manufacturing process to make new viral proteins.
Effective vaccines select recognizable antigens that induce a robust immune response. The spike protein was chosen for the mRNA COVID-19 vaccine because it is responsible for attaching to cells and gaining entry. However, research suggests that the spike protein and its S1 subunit may also be responsible for cardiovascular complications following both infection and vaccination.
![]() |
(Jaitham/Shutterstock) |
The S2 subunit may also interfere with tumor suppression, potentially explaining why COVID-19 can be more severe for cancer patients.Research shows that the spike protein is found in the blood following COVID-19 infection and vaccination. The spike protein modifies blood clotting and can stimulate an overactive immune response. A better understanding of these findings and the specific roles the spike protein and its S1 subunit play will help us determine who is most at risk for severe disease or vaccine adverse events.
Cardiovascular Effects of Spike Protein Following Infection
Although the studies are small, the spike protein has been found in the blood and clots of severely ill COVID-19 patients. The clinical evidence suggests a fingerprint of the spike protein’s cardiovascular effects.
In a study of 41 patients published in Frontiers in Immunology, 30.4 percent of the 23 hospitalized were found to have significant levels of spike protein in their circulation. None of the remaining 18 uninfected or mildly ill individuals had circulating spike protein.
A small case-control study detected the spike protein in clots retrieved from COVID-19 patients with acute ischemic stroke and myocardial infarction.
Another study detected the S1 subunit in the plasma of 64 percent of COVID-19-positive patients, and S1 levels were significantly associated with disease severity. The nucleocapsid (N) protein, a marker for COVID-19 infection, was also detected. The authors speculated that the presence of S1 and N in plasma suggests that virus fragments enter the bloodstream, potentially due to tissue damage.
The exact chain of events is not fully understood. Still, laboratory, clinical, and biopsy findings offer converging evidence suggesting a role for the spike protein and its S1 subunit in blood clotting and heart injury.
Blood Clots Associated With Spike S1 Subunit
In laboratory experiments like those performed in the Frontiers in Immunology study, the spike protein S1 subunit causes a chain reaction that sets up the right conditions for clots to form. In this chain reaction, the S1 protein binds to the ACE2 receptor on the cells lining the blood vessels. Binding to ACE2 then activates immune cells.
This domino effect can also stimulate platelet binding, increasing clotting risk. Platelets are essential clotting agents that stop blood loss following injury by clumping together. The authors further noted that in vitro, “our group recently documented that exposing sera from severe COVID-19 patients to endothelial cells induced platelet aggregation.”
In other words, the S1 subunit is of interest because, in vitro (in a test tube), it appears to cause changes to clotting mechanisms. If the S1 subunit can affect clotting agents like fibrin, complement 3, and prothrombin, this may be a mechanism through which SARS-CoV-2 can cause cardiovascular complications. Clotting causes changes in blood flow, potentially leading to thrombosis, stroke, and heart attack.
Atypical Blood Clots
Providing blood thinners to decrease the risk of clot formation did not appear to reduce the clotting risk in COVID-19 inpatients or outpatients. This may be because the clots formed after exposure to the S1 subunit may not be typical blood clots. Three findings suggest that the S1 subunit is important to clotting risk.
1. Clots Resist Normal Breakdown
First, when the S1 subunit was added to healthy blood in the lab, it created dense, fibrous clot deposits. These fibrous “amyloid” clots formed even when blood taken from healthy people was exposed to the S1 subunit.
The S1 subunit appears to be associated with clotting resistant to fibrinolysis—the normal breakdown of clots necessary to restore blood flow after injury. These amyloid clots are shown in Figure 1 below.
Amyloid clots occur when a protein is damaged and begins to fold abnormally on itself. When these abnormal amyloid proteins accumulate in the body, they can interfere with normal function.
Figure 1. Amyloid Clots Formed in Response to Spike Protein S1
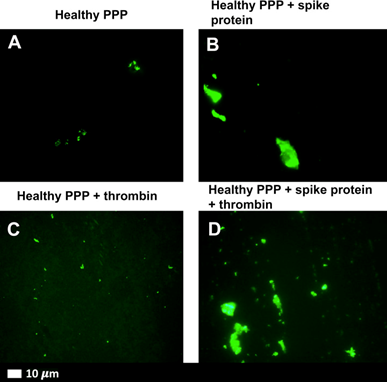
2. S1 Subunit Can Induce Amyloid Substances
Second, these dense clots may be caused by certain protein segments on the S1 subunit. The spike protein has seven protein segments (peptides) that can induce fibrous (amyloid) substances. While the fully intact spike protein (S1 and S2 subunits attached to form the full spike) did not form this amyloid, the S1 subunit did. This finding is interesting because it suggests that the subunits of the spike protein may have unique effects on cells.
3. Spike Blocks Other Clot-Inhibiting Proteins
Third, spike protein can outcompete other proteins, which prevent clots from forming. In another laboratory experiment designed to understand how this process plays out, scientists found that the spike protein blocks proteins important to breaking down clots.
In summary, the in vitro (laboratory-based) research suggests that the spike protein subunit S1 can induce clot formation and impair clot dissolution. While we do not know precisely how this translates to processes in the body, Epoch Times’ Jan Jekielek explored clotting and the role of spike protein with pathologist Dr. Ryan Cole on June 3 and Dr. Paul Marik on May 23. In the interview, Dr. Cole explained that the spike protein persists in the body longer, inflames tissues wherever it lands, and acts as an irritant or toxin in the body.
Spike Protein Found in COVID-19-Vaccinated Myocarditis Patients
Studies of COVID-19-vaccinated patients diagnosed with myocarditis found spike protein in the patients’ blood and heart muscles but not in those without myocarditis.
Found in Blood
The full-length spike protein has been found in the blood of vaccinated adolescents with myocarditis but not in the blood of those without myocarditis.
It is unclear why the spike protein was circulating freely or unbound by antibodies. The adolescents who developed myocarditis had similar immune markers to those who did not develop myocarditis. In other words, the group with myocarditis did not appear to have any immune problems.
Rather, these adolescents may have had an overactive natural immune response. Strong natural (“innate”) immunity helps the body fight off disease without any prior exposure. However, the first responders (inflammatory cytokines) can sometimes be exuberant. If the innate immune response overreacts, it may trigger myocarditis.
Found in Heart Muscle
The spike protein coded by mRNA has also been found in heart muscle cells. An endomyocardial (heart muscle) biopsy study was conducted among 15 patients with myocarditis following vaccination. No other viral infection could be found that might have caused the myocarditis.
The investigators found SARS-CoV-2 spike protein in nine of the 15 patients. Immune cells (CD4+ T) were also detected in the biopsy samples. These observations suggest an inflammatory reaction to the spike protein.
The authors concluded: “Although a causal relationship between vaccination and the occurrence of myocardial inflammation cannot be established based on the findings, the cardiac detection of spike protein, the CD4+ T-cell-dominated inflammation, and the close temporal relationship argue for a vaccine-triggered autoimmune reaction.”
A 2022 modeling study also suggests that the spike protein can cause an autoimmune response by mimicking human molecules, causing antibodies to bind to “self” proteins.
Spike S1 Detected in the Blood of Vaccinated Adults
Another study found that 11 of 13 adults vaccinated with Moderna’s mRNA-1273 had the S1 subunit in their blood as early as one day after vaccination.
Plasma was collected from 13 participants at various times during the first month after each dose. The antigens S1 and spike were measured to estimate the amount of mRNA translation into protein products.
After the first 100-microgram dose, S1 antigen was detected in the plasma of 11 participants. In contrast, the spike antigen was detected in three of 13 participants. The S1 antigen peak was detected on average five days after vaccination. Again, the timing of this peak for S1 seems to add to the clues suggesting an autoimmune response in the week after vaccination.
mRNA Detected in the Blood and Lymph Nodes After Vaccination
Vaccine mRNA, which encodes the spike protein and its S1 subunit, also persists in the blood and lymph nodes. Following vaccination, spike-encoded mRNA has been found in the blood for 15 days and in lymph nodes for up to 60 days. Spike-laden exosomes have been found circulating in the blood for up to four months. This finding is important because it refutes the CDC’s claim that the mRNA is so fragile that it dissolves quickly at the injection site (see Figure 2a in Part 1).
The lymph nodes continue creating better-fitting antibodies after any viral infection. This is a critical way that our bodies prepare for new variants naturally. However, persistently high levels of vaccine-induced mRNA and spike protein may not be helpful when the immune system is asked to respond to future variants. In other words, if the immune system is tasked with continuing to pump out antibodies to a previous variant, it may be less nimble when asked to create a high-quality antibody for a new variant.
Inadequate Clinical Trials Leave Unresolved Questions
Given what we know about the harmful effects of the SARS-CoV-2 virus, we should not have assumed that the vaccine-encoded spike protein would be harmless.
And, given what we know about clotting issues following COVID-19 infection, future studies should test whether the S1 subunit produced in response to vaccination can also cause clotting issues via the same pathway. These studies should include both lab experiments and human observations.
In addition, we do not know the relative amounts of free spike protein in circulation following infection versus vaccination.
In the case of the COVID-19 vaccines, the active ingredient was not studied prior to authorization. The manufacturers used mRNA that encodes for a substitute protein (luciferase) to test the safety and biodistribution of the mRNA vaccines.
Pfizer submitted animal biodistribution data to regulatory agencies using the surrogate RNA encoding for luciferase, as discussed in Part 1 of this series.
However, these studies were inadequate in describing how mRNA, the spike protein, its S1 subunit, and the LNP carrier would affect the human body.
In this article, we described laboratory findings showing clotting associated with the S1 subunit. Studies like these reinforce why thorough preclinical studies are so crucial. The studies conducted by pharmaceutical companies were not sufficient to address these questions.
We had very little information about how people would respond to vaccination depending on age, sex, immune status, overall health, or history of prior SARS-CoV-2 infection. The original clinical trials did not enroll enough people who had already recovered from COVID-19; they were not designed to provide an understanding of how prior infection would affect a person’s response to vaccination.
Required Pfizer Post-Authorization Safety Study Unavailable to Public
Pre-authorization studies were clearly inadequate. Post-authorization, the FDA has only acknowledged that passive surveillance is insufficient to establish safety. The agency responded to adverse event reports by requiring Pfizer to conduct additional studies, with the first monitoring report due October 2022.
On page 6 of the approval letter, the FDA acknowledges this fact (see Figure 2 below):
“We have determined that an analysis of spontaneous postmarketing adverse events reported under section 505(k)(1) of the FDCA will not be sufficient to assess known serious risks of myocarditis and pericarditis and identify an unexpected serious risk of subclinical myocarditis.
“Furthermore, the pharmacovigilance system that the FDA is required to maintain under section 505(k)(3) of the FDCA is not sufficient to assess these serious risks. Therefore, based on appropriate scientific data, we have determined that you are required to conduct the following studies. …”
Has the FDA received the monitoring report from Pfizer, which was due by Oct. 31, 2022? The next report, the interim report, will be due in October.
Figure 2. FDA Postmarketing Safety Study Requirements
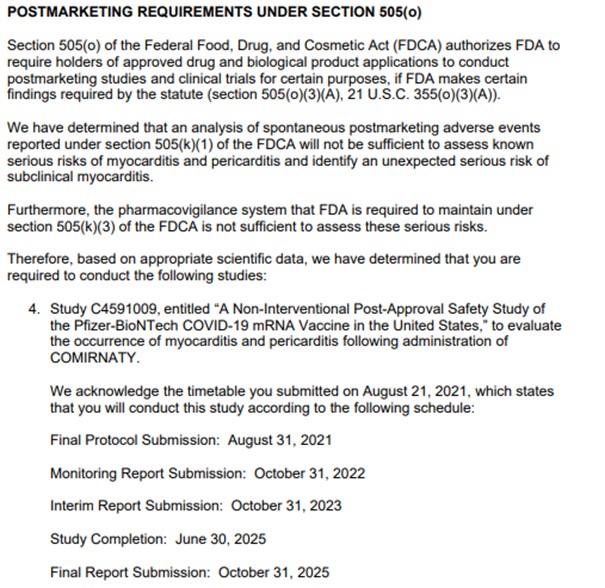
Read Part 1: FDA Overhaul Needed for New Vaccines and mRNA Therapies
Read Part 2:Health Implications of Poor COVID-19 mRNA Testing: Miscarriage, Vision Loss, Immunotoxicity
Next: In Part 5, we will discuss the mRNA manufacturing issues affecting contamination with double-stranded DNA and the potential for genome integration.
References
Addgene. Molecular Biology Reference. https://www.addgene.org/mol-bio-reference/#introduction
Alana F Ogata, Chi-An Cheng, Michaël Desjardins, Yasmeen Senussi, Amy C Sherman, Megan Powell, Lewis Novack, Salena Von, Xiaofang Li, Lindsey R Baden, David R Walt, Circulating Severe Acute Respiratory Syndrome Coronavirus 2 (SARS-CoV-2) Vaccine Antigen Detected in the Plasma of mRNA-1273 Vaccine Recipients, Clinical Infectious Diseases, Volume 74, Issue 4, 15 February 2022, Pages 715–718, https://doi.org/10.1093/cid/ciab465
Aldén M, Olofsson Falla F, Yang D, Barghouth M, Luan C, Rasmussen M, De Marinis Y. Intracellular Reverse Transcription of Pfizer BioNTech COVID-19 mRNA Vaccine BNT162b2 In Vitro in Human Liver Cell Line. Curr Issues Mol Biol. 2022 Feb 25;44(3):1115-1126. doi: 10.3390/cimb44030073. PMID: 35723296; PMCID: PMC8946961. https://pubmed.ncbi.nlm.nih.gov/35723296/
Allergic Reactions Including Anaphylaxis After Receipt of the First Dose of Pfizer-BioNTech COVID-19 Vaccine — United States, December 14–23, 2020. MMWR Morb Mortal Wkly Rep 2021;70:46–51. DOI: http://dx.doi.org/10.15585/mmwr.mm7002e1
Anderson EJ, Rouphael NG, Widge AT, et al. Safety and Immunogenicity of SARS-CoV-2 mRNA-1273 Vaccine in Older Adults N Engl J Med 2020; 383:2427-2438 https://www.nejm.org/doi/full/10.1056/NEJMoa2028436
Anderson S. CBER Plans for Monitoring COVID-19 Vaccine Safety and Effectiveness. https://stacks.cdc.gov/view/cdc/97349 October 20, 2020. Accessed 3/20/23.
Angeli F, Spanevello A, Reboldi G, Visca D, Verdecchia P. SARS-CoV-2 vaccines: Lights and shadows. Eur J Intern Med. 2021 Jun;88:1-8. doi: 10.1016/j.ejim.2021.04.019. Epub 2021 Apr 30. PMID: 33966930; PMCID: PMC8084611. https://www.ncbi.nlm.nih.gov/pmc/articles/PMC8084611/#bib0043
Baker, A. T., Boyd, R. J., Sarkar, D., Teijeira-Crespo, A., Chan, C. K., Bates, E., Waraich, K., Vant, J., Wilson, E., Truong, C. D., Lipka-Lloyd, M., Fromme, P., Vermaas, J., Williams, D., Machiesky, L., Heurich, M., Nagalo, B. M., Coughlan, L., Umlauf, S., Chiu, P. L., … Borad, M. J. (2021). ChAdOx1 interacts with CAR and PF4 with implications for thrombosis with thrombocytopenia syndrome. Science Advances. 7(49), eabl8213. https://doi.org/10.1126/sciadv.abl8213
Baumeier C, Aleshcheva G, Harms D, Gross U, Hamm C, Assmus B, Westenfeld R, Kelm M, Rammos S, Wenzel P, Münzel T, Elsässer A, Gailani M, Perings C, Bourakkadi A, Flesch M, Kempf T, Bauersachs J, Escher F, Schultheiss H-P. Intramyocardial Inflammation after COVID-19 Vaccination: An Endomyocardial Biopsy-Proven Case Series. International Journal of Molecular Sciences. 2022; 23(13):6940. https://doi.org/10.3390/ijms23136940
Bloom, K., van den Berg, F. & Arbuthnot, P. Self-amplifying RNA vaccines for infectious diseases. Gene Ther 28, 117–129 (2021). https://doi.org/10.1038/s41434-020-00204-y. https://www.nature.com/articles/s41434-020-00204-y
Chauhan, H., Mohapatra, S., Munt, D.J. et al. Physical-Chemical Characterization and Formulation Considerations for Solid Lipid Nanoparticles. AAPS PharmSciTech 17, 640–651 (2016). https://doi.org/10.1208/s12249-015-0394-x
Chui CSL, Fan M, Wan EYF, et al. Thromboembolic events and hemorrhagic stroke after mRNA (BNT162b2) and inactivated (CoronaVac) COVID-19 vaccination: A self-controlled case series study. Lancet. 2022;(50). https://www.thelancet.com/journals/eclinm/article/PIIS2589-5370(22)00234-6/fulltext
Dag Berild J, Bergstad Larsen V, Myrup Thiesson E, et al. Analysis of Thromboembolic and Thrombocytopenic Events After the AZD1222, BNT162b2, and MRNA-1273 COVID-19 Vaccines in 3 Nordic Countries. JAMA Netw Open. 2022;5(6):e2217375. doi:10.1001/jamanetworkopen.2022.17375 https://jamanetwork.com/journals/jamanetworkopen/fullarticle/2793348
daSilva RL. Viral-associated thrombotic microangiopathies. Hematology/Oncology and Stem Cell Therapy. 2011:4(2):51-59. https://www.sciencedirect.com/science/article/pii/S165838761150038X
De A, Ko YT. Why mRNA-ionizable LNPs formulations are so short-lived: causes and way-out. Expert Opin Drug Deliv. 2023 Feb;20(2):175-187. doi: 10.1080/17425247.2023.2162876. Epub 2023 Jan 1. PMID: 36588456. https://pubmed.ncbi.nlm.nih.gov/36588456/
Ehaideb, S.N., Abdullah, M.L., Abuyassin, B. et al. Evidence of a wide gap between COVID-19 in humans and animal models: a systematic review. Crit Care 24, 594 (2020). https://doi.org/10.1186/s13054-020-03304-8
European Medicines Agency https://www.ema.europa.eu/en/documents/assessment-report/comirnaty-epar-public-assessment-report_en.pdf
Faizullin D, Valiullina Y, Salnikov V, Zuev Y. Direct interaction of fibrinogen with lipid microparticles modulates clotting kinetics and clot structure. Nanomedicine. 2020 Jan;23:102098. doi: 10.1016/j.nano.2019.102098. Epub 2019 Oct 23. PMID: 31655206. https://pubmed.ncbi.nlm.nih.gov/31655206/
FDA. Considerations for Human Radiolabeled Mass Balance Studies – Guidance for Industry. https://www.fda.gov/media/158178/download May 2022.
FDA. Development and Licensure of Vaccines to Prevent COVID-19. https://www.fda.gov/media/139638/download
FDA-CBER-2021-5683-0013962 approved on: 09-Nov-2020. A Tissue Distribution Study of a [3H]-Labeled Lipid Nanoparticle-mRNA Formulation Containing ALC-0315 and ALC-0159 Following Intramuscular Administration in Wistar Han Rats. FINAL REPORT Test Facility Study No. 185350 Sponsor Reference No. ALC-NC-0552 https://phmpt.org/wp-content/uploads/2022/03/125742_S1_M4_4223_185350.pdf
Fertig TE, Chitoiu L, Marta DS, Ionescu VS, Cismasiu VB, Radu E, Angheluta G, Dobre M, Serbanescu A, Hinescu ME, Gherghiceanu M. Vaccine mRNA Can Be Detected in Blood at 15 Days Post-Vaccination. Biomedicines. 2022 Jun 28;10(7):1538. doi: 10.3390/biomedicines10071538. PMID: 35884842; PMCID: PMC9313234. https://www.ncbi.nlm.nih.gov/pmc/articles/PMC9313234/
Grobbelaar LM et al. SARS-CoV-2 spike protein S1 induces fibrin(ogen) resistant to fibrinolysis: implications for microclot formation in COVID-19 Biosci Rep (2021) 41 (8): BSR20210611. https://doi.org/10.1042/BSR20210611
Hou, X., Zaks, T., Langer, R. et al. Lipid nanoparticles for mRNA delivery. Nat Rev Mater 6, 1078–1094 (2021). https://doi.org/10.1038/s41578-021-00358-0
Iaboni A, Jindal N, Betschel SD, Song C. Second-dose mRNA COVID-19 vaccine safety in patients with immediate reactions after the first dose: A case series. Journal of Allergy and Clinical Immunology: Global. 2022;1(3): 172-174. https://www.sciencedirect.com/science/article/pii/S2772829322000200
Let’s talk about lipid nanoparticles. Nat Rev Mater 6, 99 (2021). https://www.nature.com/articles/s41578-021-00281-4
Li, JX., Wang, YH., Bair, H. et al. Risk assessment of retinal vascular occlusion after COVID-19 vaccination. npj Vaccines 8, 64 (2023). https://doi.org/10.1038/s41541-023-00661-7
Michieletto, D., Lusic, M., Marenduzzo, D. et al. Physical principles of retroviral integration in the human genome. Nat Commun 10, 575 (2019). https://doi.org/10.1038/s41467-019-08333-8
Moghimi, S. M., & Simberg, D. (2022). Pro-inflammatory concerns with lipid nanoparticles. Molecular therapy : The Journal of the American Society of Gene Therapy, 30(6), 2109–2110. https://doi.org/10.1016/j.ymthe.2022.04.011
Naturally Inspired Podcast. Jessica Rose PhD – VAERS, Data And Truth https://www.audible.com/pd/Jessica-Rose-PhD-VAERS-Data-And-Truth-Podcast/B09YMLJGBN?clientContext=132-5166709-6339436&loginAttempt=true&noChallengeShown=true
Nunez-Castilla J, Stebliankin V, Baral P, Balbin CA, Sobhan M, Cickovski T, Mondal AM, Narasimhan G, Chapagain P, Mathee K, et al. Potential Autoimmunity Resulting from Molecular Mimicry between SARS-CoV-2 Spike and Human Proteins. Viruses. 2022; 14(7):1415. https://doi.org/10.3390/v14071415
Nyström S, Hammarström P. Amyloidogenesis of SARS-CoV-2 Spike Protein. J. Am. Chem. Soc. 2022, 144, 20, 8945–8950. https://pubs.acs.org/doi/10.1021/jacs.2c03925
Ogata AF, Maley AM, Wu C, et al. Ultra-Sensitive Serial Profiling of SARS-CoV-2 Antigens and Antibodies in Plasma to Understand Disease Progression in COVID-19 Patients with Severe Disease, Clinical Chemistry, Volume 66, Issue 12, December 2020, Pages 1562–1572, https://doi.org/10.1093/clinchem/hvaa213
Ohlson J. Plasmid manufacture is the bottleneck of the genetic medicine revolution. Drug Discov Today. 2020 Oct 16;25(11):1891–3. doi: 10.1016/j.drudis.2020.09.040. Epub ahead of print. PMID: 33075470; PMCID: PMC7564888. https://www.ncbi.nlm.nih.gov/pmc/articles/PMC7564888/
Perico L, Marina Morigi M, Galbusera M, et al. SARS-CoV-2 Spike Protein 1 Activates Microvascular Endothelial Cells and Complement System Leading to Platelet Aggregation. Front. Immunol. 2022 https://www.frontiersin.org/articles/10.3389/fimmu.2022.827146/full
Qin, S., Tang, X., Chen, Y. et al. mRNA-based therapeutics: powerful and versatile tools to combat diseases. Sig Transduct Target Ther 7, 166 (2022). https://doi.org/10.1038/s41392-022-01007-w
Rafati A, Pasebani Y, Jameie M, et al. Association of SARS-CoV-2 Vaccination or Infection With Bell Palsy: A Systematic Review and Meta-analysis. JAMA Otolaryngol Head Neck Surg. Published online April 27, 2023. doi:10.1001/jamaoto.2023.016 https://jamanetwork.com/journals/jamaotolaryngology/fullarticle/2804297
Rhea EM, Logsdon AF, Hansen KM, Williams LM, Reed MJ, Baumann KK, Holden SJ, Raber J, Banks WA, Erickson MA. The S1 protein of SARS-CoV-2 crosses the blood-brain barrier in mice. Nat Neurosci. 2021 Mar;24(3):368-378. doi: 10.1038/s41593-020-00771-8. Epub 2020 Dec 16. PMID: 33328624; PMCID: PMC8793077. https://pubmed.ncbi.nlm.nih.gov/33328624/
Röltgen K, Nielsen SCA, Silva O. Immune imprinting, breadth of variant recognition, and germinal center response in human SARS-CoV-2 infection and vaccination. Cell 2022;185(6):1025-1040. https://www.cell.com/cell/fulltext/S0092-8674(22)00076-9
Rosenblum HG, Gee J, Liu R, et al. Safety of mRNA vaccines administered during the initial 6 months of the US COVID-19 vaccination program: an observational study of reports to the Vaccine Adverse Event Reporting System and v-safe. Lancet Infectious Diseases. 2022;22(6):802-812. https://doi.org/10.1016/S1473-3099(22)00054-8
Schmeling, M, Manniche, V, Hansen, PR. Batch-dependent safety of the BNT162b2 mRNA COVID-19 vaccine. Eur J Clin Invest. 2023; 00:e13998. doi:10.1111/eci.13998. https://pubmed.ncbi.nlm.nih.gov/36997290/
Singh N, Bharara Singh A. S2 subunit of SARS-nCoV-2 interacts with tumor suppressor protein p53 and BRCA: an in silico study. Transl Oncol. 2020 Oct;13(10):100814. doi: 10.1016/j.tranon.2020.100814. Epub 2020 Jun 30. PMID: 32619819; PMCID: PMC7324311.
Srinivasan M, Thangaraj SR, Arzoun H. Gene Therapy – Can it Cure Type 1 Diabetes? Cureus. 2021 Dec 19;13(12):e20516. doi: 10.7759/cureus.20516. PMID: 35004071; PMCID: PMC8723777. https://www.ncbi.nlm.nih.gov/pmc/articles/PMC8723777/
Trougakos IP, Terpos E, Alexopoulos H, et al. Adverse effects of COVID-19 mRNA vaccines: the spike hypothesis. Cell 2022;28(7): P542-554. https://www.cell.com/trends/molecular-medicine/fulltext/S1471-4914(22)00103-4
Vervaeke P, Borgos SE, Sanders NN, Combes F. Regulatory guidelines and preclinical tools to study the biodistribution of RNA therapeutics. Adv Drug Deliv Rev. 2022 May;184:114236. doi: 10.1016/j.addr.2022.114236. Epub 2022 Mar 26. PMID: 35351470; PMCID: PMC8957368. https://www.ncbi.nlm.nih.gov/pmc/articles/PMC8957368/
Wong DWL, Klinkhammer BM, Djudjaj S, Villwock S, Timm MC, Buhl EM, Wucherpfennig S, Cacchi C, Braunschweig T, Knüchel-Clarke R, Jonigk D, Werlein C, Bülow RD, Dahl E, von Stillfried S, Boor P. Multisystemic Cellular Tropism of SARS-CoV-2 in Autopsies of COVID-19 Patients. Cells. 2021 Jul 27;10(8):1900. doi: 10.3390/cells10081900. PMID: 34440669; PMCID: PMC8394956. https://pubmed.ncbi.nlm.nih.gov/34440669/
Yonker LM, Swank Z, Bartsch YC, et al. Circulating Spike Protein Detected in Post–COVID–19 mRNA Vaccine Myocarditis. Circulation. 2023:147(11). https://www.ahajournals.org/doi/10.1161/CIRCULATIONAHA.122.061025
Yu CK, Tsao S, Ng CW, Chua GT, Chan KL, Shi J, Chan YY, Ip P, Kwan MY, Cheung YF. Cardiovascular Assessment up to One Year After COVID-19 Vaccine-Associated Myocarditis. Circulation. 2023 Aug;148(5):436-439. doi: 10.1161/CIRCULATIONAHA.123.064772. Epub 2023 Jul 31. PMID: 37523760; PMCID: PMC10373639.
Zheng Y, Zhao J, Li J, Guo Z, Sheng J, Ye X, Jin G, Wang C, Chai W, Yan J, Liu D, Liang X. SARS-CoV-2 spike protein causes blood coagulation and thrombosis by competitive binding to heparan sulfate. Int J Biol Macromol. 2021 Dec 15;193(Pt B):1124-1129. doi: 10.1016/j.ijbiomac.2021.10.112. Epub 2021 Oct 29. PMID: 34743814; PMCID: PMC8553634. https://www.ncbi.nlm.nih.gov/pmc/articles/PMC8553634/
About the Authors:
Allison Krug is an epidemiologist and program manager with experience leading population health programs. She's the lead author of the first stratified risk-benefit analysis of mRNA vaccination among adolescents and editor for 400+ research manuscripts published in high impact factor, peer-reviewed journals. She's also the founder and CEO of Artemis Biomedical Communications, LLC.
Dr. Duriseti is a clinical associate professor and physician-scientist at Stanford University. He received his M.D. from the University of Michigan and his medical training and Ph.D. in Computational Decision Modeling from Stanford University. He has practiced in clinical Emergency Medicine in both community and academic settings for over 22 years.
Dr. Xiaoxu Sean Lin is an assistant professor in the Biomedical Science Department at Feitian College in Middletown, N.Y. Dr. Lin is also a frequent analyst and commentator for Epoch Media Group, VOA, and RFA. He is a veteran who served as a U.S. Army microbiologist and also a member of Committee on the Present Danger: China.
Yuhong Dong, M.D., Ph.D., is a senior medical columnist for The Epoch Times. She is a former senior medical scientific expert and pharmacovigilance leader at Novartis Headquarters in Switzerland, and was a Novartis award winner for four years. She has preclinical research experience in virology, immunology, oncology, neurology, and ophthalmology, and also has clinical experience in infectious disease and internal medicine. She earned her M.D. and a doctorate in infectious diseases at Beijing University in China.
Comments
Post a Comment